The Future of Chronic Pain
For the millions of people suffering with daily physical pain, nerve stimulation and gene editing offer new hope beyond addictive opioids.
Pain is a classic human health problem in search of a good medical solution. It is widespread, a leading cause of disability, and a major burden to economies worldwide. Chronic pain—defined as pain that persists for longer than 12 weeks despite medication or treatment—is estimated to affect some 20 percent of adults in the world (or 1.5 billion people), with hundreds of millions more being newly diagnosed with it each year. Clinically, chronic pain can be extremely difficult to manage, not least of all because it often manifests itself in the absence of physical injury.
Currently, it is most often treated with synthetic opioid analgesics, and this has caused a now tragically well-known health crisis. In 2020, more than 90,000 Americans died from opioid overdoses, and opioid addiction is also a major driver of U.S. suicides, which have risen over the last two decades. The U.S. Centers for Disease Control and Prevention estimate that prescription opioid misuse has a total economic burden of nearly $80 billion in the United States alone—a figure that includes health care, loss of productivity, treatment for addiction, and involvement of the criminal justice system.
The potential market for pain relief is also enormous. Global sales of opioids hit $18.5 billion in 2018 alone and are projected to grow by more than 3 percent per year, reaching $22.4 billion by 2026. This underlines the fact that so many people are so desperate for prescription analgesics that they are still driving sales up despite all the unwanted and dangerous sedative and addictive side effects of the drugs, a decade into the opioid epidemic. The numbers also explain why developing and selling prescription drugs for pain is a crowded field full of major pharmaceutical players—and why relieving pain is the stuff drug industry dreams are made of.
A small-molecule drug that could alleviate this suffering as effectively as opioids but more safely, and with fewer side effects, would be a blockbuster. Developing such a compound is easier said than done, however. Most of the clinical trials for pain drugs over the last two decades have either fallen flat in unfulfilled promises or been shut down early from outright failure. New strategies for treating pain are desperately needed, and the rewards will be rich for the companies that succeed.
Walk a mile over burning coals
Recent advances in pain research have opened up several promising new avenues. One line of research in particular—genetic studies of families with an inherited insensitivity to pain—may pave the way for the development of new strategies for pain relief. In the past 15 years, these individuals have provided some valuable insights into the molecular mechanisms of pain, suggesting ways to develop novel analgesics using a traditional drug pharmacology approach and, more recently, based on newly developed gene-editing techniques.
Inherited congenital insensitivity to pain is an extremely rare condition and is only believed to occur in fewer than one in a million people. Until the late 1950s, just 46 such cases had been described in the medical literature. Since the turn of the century, however, researchers have identified several extended “consanguineous” families, members of which share this unusual characteristic, and have also mapped the genes and variants that cause it.
Pain serves the evolutionarily vital function of alerting us to potentially life-threatening injuries.
One famous case is that of a 10-year-old child from northern Pakistan who, according to the 2006 case study that identified the underlying mutation, was “well known to the medical service” after regularly performing a twisted type of street theater in which he “placed knives through his arms and walked on burning coals, but experienced no pain.”
Such a superpower is fraught. Pain serves the evolutionarily vital function of alerting us to potentially life-threatening injuries, and people who are unable to feel pain tend to be highly accident-prone, often dying in childhood as a result of injuries or illnesses that go unnoticed. By four years of age, they typically exhibit serious injuries to their tongue and lips caused by biting themselves, and go on to experience frequent cuts, bruises, and fractures. The Pakistani street performer tragically died at the age of 13 after jumping off a house roof, according to the account by scientists who described his case.
Reduce the pain in the brain
Studies of such individuals and affected family members have identified the SCNA9 gene as a key player in the transmission of pain signals from the body to the brain. This gene encodes the component pieces of a sodium channel called Nav1.7, which forms a membrane-spanning pore in the endings of pain-sensing nerve cells—also known as nociceptors—and elsewhere. In sensory neurons, Nav1.7 is essential for generating the nervous impulses that carry pain-related information up into the spinal cord and then to the brain. Without it, these signals cannot be generated. Various mutations within the SCNA9 gene cause the synthesis of non-functional Nav1.7 molecules; as a result, people who inherit any of these mutations feel no pain.
Nav1.7 had already been implicated as being important for pain signaling before this mutation had been identified. In 2004, a team of researchers at University College London reported that genetically engineered “knock-out” mice lacking the SCNA9 gene have a higher threshold for mechanical and thermal pain, and also exhibit markedly reduced, or completely abolished, responses to inflammatory pain. Subsequently, researchers at Yale School of Medicine reported another human SCNA9 mutation that produces an overactive Nav1.7 channel. This mutation causes erythromelalgia, or “man on fire” syndrome, another rare inherited condition in which warm temperatures and moderate exercise trigger intense, burning pain in the extremities.
This research set off an industry-wide hunt for novel analgesics that act on Nav1.7. Yet after 15 years of effort, the search for new painkillers has proven unsuccessful. Many molecules that target Nav1.7 have been discovered, but most of them have failed in testing because they also inhibit other sodium channel sub-types, which are found throughout the body, and play fundamental roles in a wide variety of physiological functions, including regulating muscle contraction and cardiac rhythm. The lack of specificity produces side effects that make the compounds unfeasible as analgesics, and in the past 5 years, various pharmaceutical and biotechnology companies have discontinued trials of small-molecule Nav1.7 inhibitors.
In the past 20 years, the discovery of another, unrelated group of molecules has revolutionized our understanding of pain. TRP channels are also found in nociceptor nerve endings, where they act as sensors for temperature and a wide range of noxious chemicals. TRPV1, for example, is a “hot” pain receptor that becomes activated by temperatures of 108°F and higher. The receptor can also be activated by capsaicin, the spicy chemical found in jalapeños and pepper spray. So the same protein that generates pain-related signals when we touch a hot surface is also responsible for the burning sensation we feel when eating spicy food. At the other end of the scale, the TPM8 channel is activated by ambient temperatures dropping below 79°F, and also by menthol, which is found in peppermint. That’s why we feel a slight chill when the wind blows on a mild spring day—and why our brain associates minty gum with a cool blast.
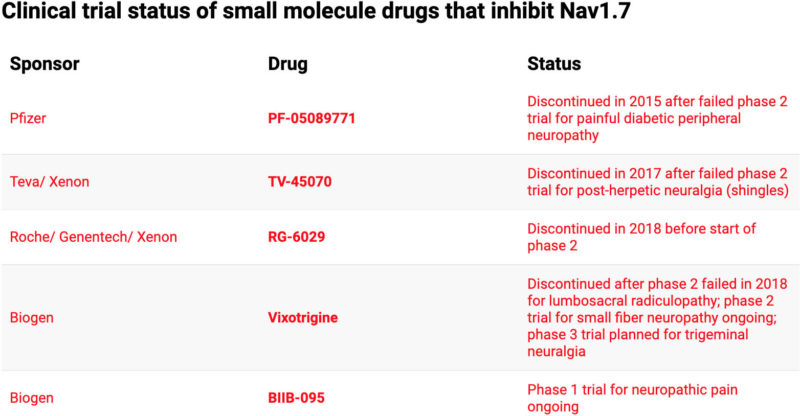
Whereas Nav1.7 is expressed in all pain-sensing neurons, different TRP channels (or combinations of them) are found in subsets of pain-sensing neurons, making each subset sensitive to a specific temperature range. TRP channels comprise a “superfamily” of related proteins that are found throughout the animal kingdom, where they serve a range of functions. Vampire bats have evolved the ability to use TRPV1 to detect infrared heat signatures in order to bite a blood vessel. Pit vipers, boas, and pythons have the most sensitive of all known TRPV1 channels, which enables the infrared vision that helps them hunt small, furry creatures at night.
In humans, TRP channels are known to play important roles in neuropathic and inflammatory pain, and mutations in one of them are associated with a rare inherited disorder called familial pain syndrome.
The discoveries of these mutations prompted a search for new painkillers, which has been slightly more successful than the experimental drugs targeting Nav1.7, but still has not produced any approved drugs. A number of small-molecule drugs that modulate TRP channels have been identified, and given the diverse roles of TRP channels in the body, drug discovery efforts targeting these channels have recently been expanded beyond pain management into other disease areas, including asthma, cancer, and metabolic disorders. But progress is slow, with few candidates making it to clinical trials, and none as yet approved. A better understanding of TRP channel biology will likely be needed to accelerate the discovery of any new drug treatment.
CRISPR take me away
More recent research suggests that newly developed genome editing techniques could one day be used to treat pain. Scientists at the University of California, San Diego, are using CRISPR to deliver a gene encoding a “suppressor” protein, which binds to the SCNA9 gene, preventing synthesis of Nav1.7 channels. Delivery requires the use of viral vectors to carry the genes into cell nuclei; in this case, though, binding of the suppressor protein is reversible, and so the SCNA9 gene is temporarily silenced rather than permanently altered.
In a study published in the journal Science Translational Medicine earlier this month, the team reported that this genetic construct effectively produces long-lasting relief from several different types of pain when injected in mice.
“You wouldn’t want to permanently lose the ability to feel pain, [so] we’re not doing something irreversible,” says lead researcher Ana Moreno. “It’s not cutting out any genes, so there are no permanent changes to the genome.”
Moreno and her collaborators founded the preclinical stage startup Navega Therapeutics to develop the technique. They plan to try using it to treat inherited erythromelalgia and, eventually, more common forms of chronic pain.
It’s unlikely that this approach can be applied to humans any time soon, however. “The big problem is that making these viruses for humans costs millions of dollars, so I can’t believe that this will take off for a very long time,” says John Wood, head the University College London lab in which the SCNA9 knock-out mice were first created—though he was not involved in the research. Even once we have created the biotech, doing the clinical trials is even more expensive, will take years to complete, and faces the sobering reality that more drugs fail in human trials than succeed. And besides the cost, the technique would likely have unwanted side effects: “Nav1.7 is also found in the pancreas, where it plays a role in insulin secretion,” says Wood. Silencing the human SCNA9 gene could, therefore, have deleterious effects, and would require rigorous proof-of-concept studies in terminally ill patients. “That’s hugely problematic, in terms of both cost and ethical approval.”
Electrical medicine rubs the pain away
A recent phase 2 clinical trial provides the strongest evidence yet that a technique called transcutaneous electrical nerve stimulation (TENS) can alleviate pain for people with fibromyalgia, a complex, long-term condition characterized by widespread musculoskeletal pain accompanied by fatigue. Analgesics are only modestly effective in treating fibromyalgia, and clinicians are increasingly recognizing that non-pharmacological means like TENS are likely to be more effective and should be considered first.
The trial, carried out by researchers at the University of Iowa and Vanderbilt University Medical Center, recruited 300 women diagnosed with fibromyalgia and randomly divided them into three groups. Members of one group used a TENS device at home for two hours a day for four weeks, alongside their regular treatment of light exercise. (The small battery-operated device delivers mild electrical currents via electrode pads attached directly to the skin.) Members of the second group used a sham device that delivered milder, non-therapeutic currents for the same period, while members of the third group continued with just their regular treatment. Before, during, and after the intervention, all of the participants filled out questionnaires designed to gauge their perceived pain.
The results are encouraging. Those who had received TENS reported a significant reduction in both movement-related pain and fatigue. Almost half of them reported at least a 30 percent reduction in pain, compared to less than one quarter of those who received sham TENS, and just 14 percent of those in the third group, who did exercise alone. As for side effects, fewer than 1 in 20 of the participants who received TENS reported experiencing any pain or irritation from the electrodes.
The results suggest that TENS had a lasting effect on the people who received it, showing greater reductions in pain after four weeks of home use. And while previous research had suggested that repeated use of TENS becomes less effective with time, in this trial, the method remained effective after eight weeks. (A related method, called spinal cord stimulation, also shows promise, but requires surgery to implant a “pacemaker” device into the spinal cord.)
Transcutaneous electrical nerve stimulation is not new. It has been used to treat various kinds of chronic pain, including arthritic pain, period pain, sports injuries, and neck and back pain since the 1970s. There have, however, been very few large, well-designed trials testing the effectiveness of the method.
“TENS alleviates movement-evoked pain, which is critical because it means that patients can move around while applying it.”
“There’s a long-standing unresolved controversy about whether TENS works or not,” says Mark Johnson, a professor of pain and analgesia, and director of the Centre for Pain Research at Leeds Beckett University in England. “Most of the trials have fewer than 100 participants, and as a consequence it’s easy to overestimate the effects of the treatment.” But he called the Iowa and Vanderbilt study, which he was not involved with, the most robust clinical evidence to date. “It demonstrates that TENS alleviates movement-evoked pain, which is critical because it means that patients can move around while applying it.”
Johnson explains that TENS works in the same way as rubbing a painful body part. “When you rub, you’re activating non-noxious nerve fibers, and this inhibits pain-related information from getting to the brain,” he says. Similarly, TENS passes electricity through the skin and artificially activates those same fibers. “It electrically rubs the pain away,” Johnson says.
It also appears to be safe. “There’s virtually nothing in the literature about adverse events [other than the] electrodes causing irritation or itchiness,” he says. “Big pharma is looking at TENS as a way of trying to mitigate the challenges they have due to the opioid crisis, paracetamol [Tylenol], and other non-steroidal anti-inflammatories. They all have quite toxic side effects, and often aren’t as clinically efficacious as you’d like to believe.”
Johnson adds that he and his colleagues are about to publish the largest meta-analysis of TENS research to date, including almost 400 clinical trials, which shows that the method does indeed reduce pain intensity more effectively than placebo. This could, he says, “draw a line under the efficacy argument,” eventually leading to approval of more TENS devices for specific types of pain. Meanwhile, pharmaceutical and biotech companies continue on their quest to discover and test new painkillers—so there is hope for the hundreds of millions of people worldwide living with chronic pain.