Bit.bio’s new approach for reprogramming pluripotent stem cells could standardize the reliable, large-scale manufacture of cells for research and therapy.
The hopes and dreams of regenerative medicine and the use of stem cells to treat or cure diseases have been a beacon of promise for 25 years. But after a roller coaster ride of scientific breakthroughs, clinical setbacks, policy wrangles, and an explosion of suspiciously charlatan clinics pushing unproven treatments, we are mostly still waiting for those cures.
Now bit.bio, a synthetic biology company focused on human cells and headquartered in Cambridge, England, has presented new data at the 2023 annual meeting of the International Society for Stem Cell Research (ISSCR) conference last week in Boston that showed they can engineer stem cells with what they are calling an unparalleled level of consistency. Company officials say their achievements in the last few months will set a new standard for the manufacture of human cells, calling their innovation so disruptive they claim it will do for stem cell biology what CRISPR has done for genetics.
Bit.bio’s achievement builds on the pioneering work of Shinya Yamanaka, a professor at Kyoto University in Japan and a senior investigator at the Gladstone Institutes in San Francisco, who discovered how to transform ordinary mature adult skin cells into more primitive precursor cells, which look and act like the sort of embryonic stem cells found in the early states of embryonic development in the womb.
Yamanaka announced his original breakthrough in 2006 after inserting four genes into adult skin cells in mice. These four genes encoded a set of proteins called transcription factors, which regulate the activity of other genes in a cell. By inserting them into the mouse cells, he was able to transform those cells, rolling back their development and inducing them into a naïve, undifferentiated state. From there, those cells he created in the laboratory were potentially capable of growing into whatever new mouse cell they could be directed to become. A year later, Yamanaka announced he had done the same thing with adult human skin cells, transforming them into what he dubbed “induced pluripotent stem cells” (iPSCs), which were similar to the embryonic stem cells discovered by James Thomson of the University of Wisconsin Stem Cell and Regenerative Center 25 years earlier.
In 2012 Yamanaka won the Nobel Prize in Physiology or Medicine for this discovery because the iPSCs were immediately recognized to hold great promise for the emerging field of regenerative medicine because they represented a single source of cells that could develop into any cell in the human body and be used to replace cells lost to the ravages of diseases like dementia or diabetes.
Yet despite the enthusiasm and high hopes of the scientific community that the discovery would translate into thousands of new therapies, that has still not happened yet—something that bit.bio aims to change with its new technology.
So far, only one success
Regenerative medicine belongs to a broader category of cell-based medicine, and as of 2023, the only cell-based treatments that are approved by the U.S. Food and Drug Administration (FDA) for any disease are the so-called CAR T-cell therapies for the treatment of certain blood cancers, including lymphomas, some forms of leukemia, and, most recently, multiple myeloma.
CAR T therapy (an acronym for chimeric antigen receptor T lymphocyte-cell therapy) is not a type of regenerative medicine at all but a type of immunotherapy in which T cells are harvested from a person, genetically engineered to target the specific antigens found on the surface of the cancer cells, and then reintroduced back into the body to seek and destroy those cancer cells carrying that antigen. The treatment has produced dramatic results for many people and in some cases has eliminated all evidence of cancer. But the cost of CAR T therapy is high— between $500,000 and $1,000,000 per person, according to the Chronic Lymphocytic Leukemia Society. And the risks of adverse side effects are serious enough that just this week the FDA put a phase 2 clinical trial of Gilead Sciences’ new CAR T therapy for multiple myeloma on hold because of a patient death in the study—only months after the company went all in with a $225 million dollar investment.
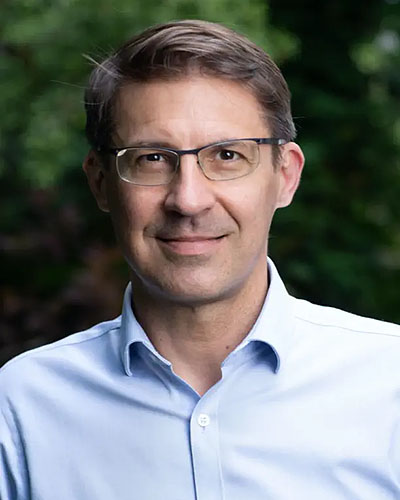
“There is no question that CAR T treatment is a significant breakthrough,” says bit.bio CEO and founder Mark Kotter, a University of Cambridge neurosurgeon. But it is limited, he says, because each treatment requires collecting and manipulating cells from the specific person being treated. That means those cells will not be useful to anyone else—and even then, tragically and inexplicably, CAR T therapy only works in roughly one third of patients.
For the last few decades, the hope has been that iPSCs could be used to manufacture a broader source of cells for therapies like CAR T, making them available at larger scale to more people and at lower cost. But so far that hasn’t happened, “despite 25 years of research,” says Marius Wernig, co-director of the Institute for Stem Cell Biology and Regenerative Medicine at Stanford University. Wernig pioneered the reprogramming of stem cells into brain cells and is a member of the scientific advisory board of bit.bio.
The fact that iPSCs are hard to work with and difficult to produce at scale, Wernig says, has been the biggest barrier in regenerative medicine. “I believe that the one single reason why there is not a clinical treatment based on iPSCs is the difficulties converting them into relevant cell types,” Wernig says.
For example, to treat Parkinson’s you would need dopaminergic neurons, the specific type of brain cell that degenerates in the disease. “While this is possible on a small scale with traditional differentiation, it has been a challenge to industrialize the manufacture,” Wernig says. “This is also true, for example for pancreatic islet cells (to cure diabetes), which made big news a few years back.”
Unparalleled consistency
Wernig is part of the team of researchers who presented the new data from bit.bio at the stem cell meeting in Boston last week. The company’s “opti-ox” technology (an acronym for optimized inducible overexpression) borrows from Yamanaka’s work and goes further, they say, by targeting what are called genomic safe harbor sites, which are regions of the genome that maintain transgene expression without disrupting the function of host cells. Targeting those safe harbor sites, the company says, enables the direct reprogramming of millions of human induced pluripotent stem cells at a time into highly defined and mature human cell types, and the company claims it can do so more robustly than ever before.
“This technology can speed up the manufacturing process by a factor of 10 and lower costs by two orders of magnitude.”
Bit.bio says in its press release that it is “industrializing human cell manufacturing.” What has limited the potential of iPSC therapy up to now is that the cells produced tend to be inconsistent. Batches of differentiated cells often contain all the cells you want plus many you don’t, which severely limits the ability to use these cells in the clinic. But the company claims they tested samples of cells selected from different manufacturing lots, and they showed fewer than 1 percent difference in terms of gene expression with other lots. “This achievement sets a new standard for the manufacture of human cells,” their press release states.
The new technology can also speed up the manufacturing process by a factor of 10, Wernig adds. “And if you look at the costs of today’s cell therapies, it should be able to lower these by two orders of magnitude.”
One outside expert who is not involved with the company but familiar with its work agreed that bit.bio has the potential to transform the field of stem cell medicine.
Vittorio Sebastiano, a professor at the Stanford Maternal & Child Health Research Institute, says the results are exciting and promising. “Such a degree of consistency is instrumental for some specific applications of technology, like drug screening and generation of uniform types of cells for example in the food industry (for artificial meat),” he says. More studies are needed to see how the consistency and reproducibility improves regenerative medicine. In the body even seemingly identical cells can be heterogenous in their gene expression, responding differently to chemical cues in the body depending on where they are transplanted. “It will be interesting to see whether such ‘uniformity’ may be truly needed for regenerative medicine,” Sebastiano says.
Bertie Göttgens directs the University of Cambridge Stem Cell Institute, which partners with bit.bio and other companies in its annual forum. “Cambridge has a proud track record in stem cell research with Nobel prizes for IVF, embryonic stem cells, and reprogramming. Bit.bio has grown out of this Cambridge ecosystem to be a serious contender in the cell programming R&D landscape,” he says. “Their recent reports on cell programming robustness and efficiency are going a long way towards establishing bit.bio as an international leader in this competitive field.”
Producing almost identical cells at scale, with consistency, and precision means introducing a solid manufacturing paradigm into cell biology, says bit.bio CEO Kotter. “As solid, I would say, as you’d see in conventional engineering disciplines,” he says, adding, “I believe that bit.bio is poised to become a cornerstone in the upcoming regenerative medicine revolution.”
The promise of industrial cell biology
Kotter says he turned to human stem cell research because of his ambition to create cell therapies and his frustration with the drug development process. “We know that most drugs in clinical trials fail,” he says.
“We now have a technology that will lead to the development of novel treatments in the next five years for neurodegenerative and other serious illnesses.”
One type of therapy that may benefit from the new technology are CAR T cells for cancer. Many tumors are resistant to this treatment because the cancer spreads to areas the cells don’t reach or the body counters the activity of the CAR T cells. “Only 30 percent of eligible cancer patients respond to immunotherapies,” Kotter says. The reason for this failure, he believes, is because of inconsistent cell preparations, which is what bio.bit’s platform was designed to fix by providing consistency across all cells. “Our technology has solved that problem with consistency,” he says—though he admits not all immunologists agree.
Alzheimer’s is another area of interest to bit.bio. Kotter says the reasons for failure in dementia drug development are many, but one important one is the overreliance on animal models. “There is no mouse in the world that has Alzheimer’s. So, the best scientists could do was to use genetically modified mice to mimic the disease. But mice are not people. Developing drugs in mutated mice rarely works,” he says. The bit.bio platform could potentially generate better human cell lines for screening new drugs, although any potential drugs found would still have to prove safe and effective in clinical trials.
In 10 years Kotter hopes to have a wide pipeline of regenerative medicines in clinical trials, with some of them already available in the clinic. “We could have 3D-printed organ systems such as those of Martine Rothblatt’s company United Therapeutics Corporation,” he says.
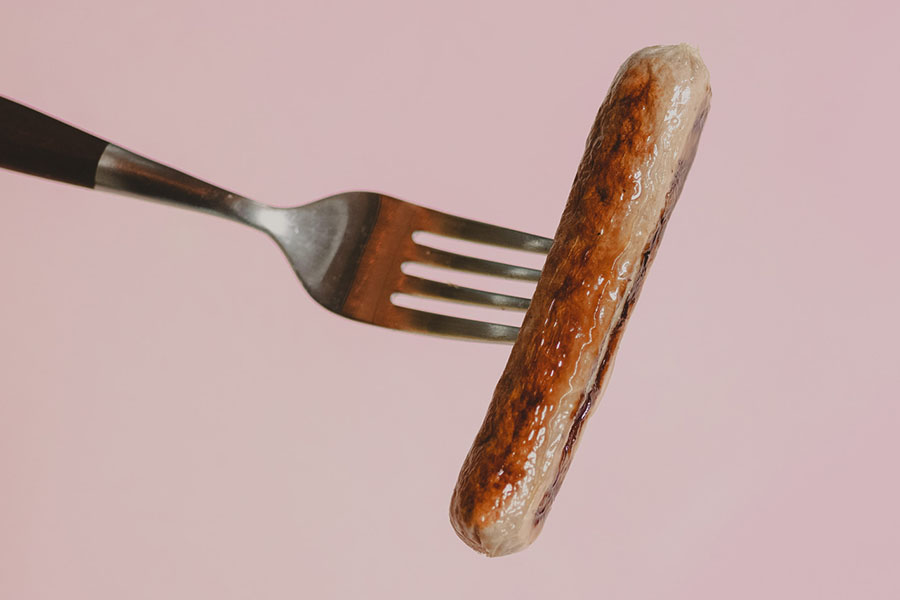
Proto.life spoke with Roger Pedersen of Stanford University School of Medicine who chairs bit.bio’s scientific advisory board. A developmental and stem cell biologist, he made national news when he relocated to England in 2001 due to the debate over limiting federal research involving embryonic stem cells that took place during the Bush administration. In 2018, he relocated back to the United States.
Pedersen is excited about bit.bio and believes its technology will help break the logjam that has prevented treatments based on iPSC therapies. “For the first time since the initial publication 25 years ago on human pluripotent stem cells, we now have a technology that will lead to the development of novel treatments in the next five years for neurodegenerative and other serious illnesses,” he says.
The company’s opti-ox technology is also being used to produce cultured meat at Meatable, a company Kotter co-founded in Leyden, the Netherlands. A recent tasting in Singapore featured a range of the company’s pork products, including sausages and Chinese dumplings, which will reach price parity (with commercial products) at $10 per kilo. “Meatable is on track both scale-wise and cost-wise,” Kotter said, “providing products that taste good and are better for the planet.”
Kotter says his overall goal is to democratize access to human cells, eliminating high costs and inconsistencies. “We have developed a platform in bit.bio that should be able to manufacture any cell type mapped in the Human Cell Atlas,” he says. “I want to make these available to scientists, and more importantly patients who could benefit from the transformative impact of cell therapies.”