With billions of dollars flooding into longevity, what role will epigenetic clocks play in measuring and intervening in aging?
Steve Horvath doesn’t like the term “anti-aging.” The father of modern epigenetic clocks has sparked a booming new field designed to slow, stop, and even reverse aging. Google the term “anti-aging,” and you’ll get pages of results still crowded with wrinkle creams, supplements, and serums—so it’s not hard to understand why Horvath shudders a bit at the phrase.
When Horvath first described epigenetic clocks, scientists began to speculate that altering them could reverse aging. After all, if certain patterns of DNA methylation at certain sites in cells in certain tissues of your body are hallmarks of aging, could shifting them somehow reverse aging?
The short answer: It’s possible.
Horvath’s clock predicts biological age based on activity at a selection of DNA methylation sites, which regulate gene expression. Somewhat like a volume dial, turning on or off various sites can dial up or down gene expression. It’s a complex process, with millions of sites in total.
In Horvath’s case, he found that the action of 353 sites, considered together, yielded an exceptionally accurate test for chronological age. Using data from 8,000 samples from 82 DNA methylation datasets compiled by other researchers, Horvath examined patterns of methylation across 51 tissue and cell types. With those datasets, Horvath developed a biomarker-based clock for aging, then proved its accuracy. In practice, any two 25-year-olds would have the same chronological age, but the Horvath clock might put one at 20 and another at 30, based on individual DNA methylation patterns. The implication, if yet unproven, is obvious: People aging faster could die younger and have less healthy lives.
Horvath is at the center of a well-funded race to answer questions related to epigenetic clocks. He hopes to harness those clocks and other offshoots of his findings as a principal investigator at the San Diego campus of Altos Labs. There are so many possibilities, he says, that one career isn’t time enough to research them all. For now, he is focusing on tinkering with epigenetic age as a means to slow aging, backed by the $3 billion funneled by Altos investors and an all-star team that includes Nobel laureate Jennifer Doudna, who co-invented the CRISPR genome editing tool, and Shinya Yamanaka, another Nobel prize winner who discovered a way to transform differentiated cells into a stem cell-like state by manipulating four gene regulators now known as Yamanaka factors.
“This thing of us aging and getting wrinkly is really a problem of our generation, and sooner or later this will be solved,” Horvath says.
“If something turns out to be a small-molecule drug, we might be able to produce it literally for cents.”
But so-called anti-aging or rejuvenation techniques could do much more than reverse wrinkles for a chosen few Silicon Valley investors. In fact, Andrew Steele, author of Ageless, a book on the science of preventing and reversing aging, says epigenetic therapies stand to help lower-income nations, where people have shorter average lifespans, more than wealthier ones.
Steele points out that even therapies funded by billionaires must undergo clinical trials with tens of thousands of participants. And pharmaceutical therapies from those trials, surely, would be available to the general public for a price. Some potential therapies, like receiving injections of stem-like cells that help shift the body’s epigenetic programming to a younger state, could be quite expensive. But “if something turns out to be a small-molecule drug, we might be able to produce it literally for cents,” Steele says. “You’ve got to hope that governments would subsidize these things and that the pharmaceutical companies produce them as cheaply as possible if they turn out to have such widespread benefits.”
“The first benefits we will see, hopefully, or what we all are aiming for is that people are just healthier at old age and don’t have to suffer as much,” says Tamir Chandra, a systems biologist at the University of Edinburgh in Scotland. That could lessen the financial burden of eldercare. From a more cynical perspective, therapies that stave off frailty and disability in our final decades have an economic benefit: more productive working years.
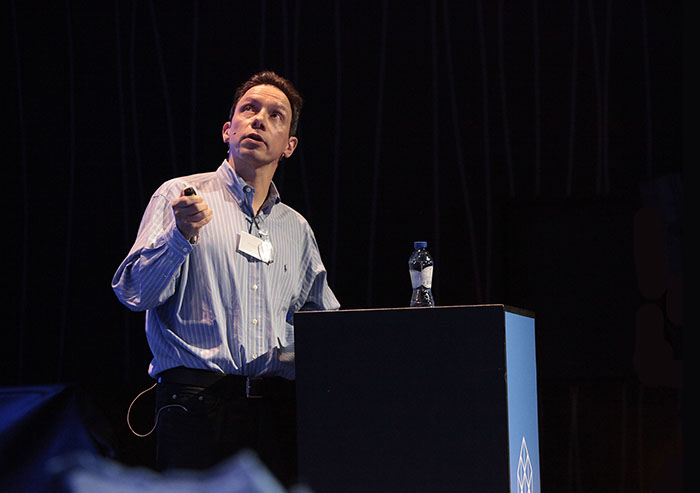
Epigenetic clocks: Just a marker of time?
Despite their obvious promise and growing popularity, epigenetic clocks still have some notable shortcomings. First, it’s difficult to tell exactly how accurate biological age measurements are. According to one expert, epigenetic clocks are much better at predicting lifespan than previous techniques, like oxidative damage or telomere length. But the challenge with longevity research is that studies to determine whether biological age predictions translate to actual lifespans take decades. In other words, if you’re 25 with a biological age of 30, will you die five years sooner than average? Secondly, scientists haven’t pinpointed which changes are directly caused by aging. It’s possible that some changes occur by happenstance in older people, independent of aging. In other words, some changes we associate with aging may not actually impact the length or quality of our lives. Finally, scientists like Chandra suspect that epigenetic clocks are more of a measure of biological age than a driver of it.
“Think of the clock as a wristwatch. If you broke your wristwatch, the time would still go on,” he says. “My guess would be that if we stopped these [methylation] sites from changing, we wouldn’t interfere much with the aging process.” But Chandra still sees epigenetic clocks as an excellent marker for biological age, a measure of how quickly the aging process is proceeding in humans or other animals, independent of calendar years. For example, a smoker at age 50 might have an epigenetic age of 65, while a person at the same who exercises frequently might have an epigenetic age of 45.
Horvath is a bit more optimistic. “I would say there are locations that actually matter a lot,” he says of the DNA methylation sites that drive his clock. “If you change the right locations, you may actually rejuvenate cells. I won’t claim that, I’m just saying nobody knows.”
Horvath’s clock is considered highly reliable for chronological age. And it’s been followed by dozens of others, though Horvath says it doesn’t matter what clock you use. Thanks to machine learning, each epigenetic clock measures DNA methylation rates at so many sites that their results tend to be the same.
There have been upgrades that hone in on healthspan, like the DNAm PhenoAge, a second-generation clock that predicts the risk of aging-related events like physical functioning, cancer, or Alzheimer’s. Morgan Levine developed it as a postdoc in Horvath’s UCLA lab; this year, she joined him at Altos Labs. Building on Levine’s finding, Horvath created GrimAge, another second-generation clock that predicts age-related disease and decline.
There’s one caveat. Different anti-aging or rejuvenation interventions—especially those targeted to a single tissue type—may impact the various DNA methylation sites differently. In these cases, Horvath says, “you really want to think very carefully which clock to use.” Measuring the impacts of exercise on muscle cells is one example.
“I don’t think the clock is extremely good at tracking environmental stimuli,” says aging researcher Nir Eynon, whose research at the University of Victoria in Australia focuses on epigenetic shifts caused by exercise. He studied methylation rates in skeletal muscle biopsies, looking for age- and exercise-related pattern shifts.
The Horvath clock didn’t include muscle cells in its initial machine learning analysis and, according to Eynon’s analyses, it didn’t seem to be responsive to exercise. As an expert in exercise and aging, Eynon says he can “almost guarantee” an epigenetic benefit from an active lifestyle. He soon developed a clock for human skeletal muscle that honed in on epigenetic benefits of exercise; Horvath co-authored the study.
So, over time, scientists hope to develop ever more intricate, accurate, and explainable epigenetic clocks whose results could be interpreted not just as reliable clues to the rate of aging, but more definitive designations of how the body is responding to various stressors.
A big question remains. Do we need individual epigenetic clocks for each bodily system? Or is a systemic, multi-tissue clock better? “That’s a big question I’m asking myself every day,” Eynon says. For now, he’s working with a new clock that incorporates tissue data from 15 different organs, recognizing the widespread benefits of exercise: “Every healthy intervention is systemic.”
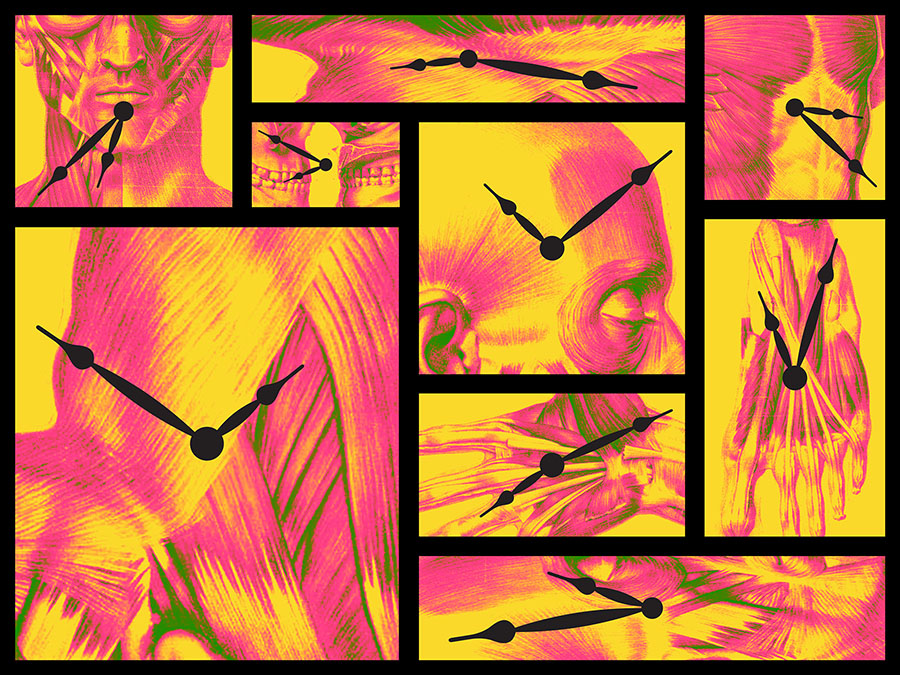
Rejuvenation is in our DNA
Aging is surprisingly ill-defined. Scientists don’t agree exactly why we age or how aging evolved. Current ideas include an increased chance of dying, loss of function, accumulation of damage over time, continued development, age-related changes, or now, an increase in biological age as measured by epigenetic clocks. Despite the lack of consensus, “late in life, they all apply, and they all go hand in hand,” says Harvard biomedical researcher Vadim Gladyshev. Still, “there must be one, most important feature that defines aging, but there is currently no consensus on what this feature is,” he adds.
Any type of lifespan-lengthening intervention requires some way of measuring rejuvenation. But there’s no consensus on which epigenetic clock would be best to measure the reversal of aging, according to Gladyshev. “The clocks are built to quantify the transition from young to old,” he says. “Going from old to young is not necessarily the same trajectory.”
Yet, Gladyshev’s work has already unearthed one natural type of rejuvenation that occurs in embryos. It started with measuring the epigenetic age of embryos using various aging clocks. Unsurprisingly, human embryos clocked an age of just above zero. So, how do cells from aging donors produce an embryo with an age of nearly zero? Gladyshev, wondering how this was possible, pinpointed the moment at which the embryo altered its methylation and reset its age to nearly zero by studying the process in mice and human embryos. It took place shortly after the embryo implanted on the lining of the uterus, but the mechanism behind this seeming rejuvenation remains a mystery.
Epigenetic clocks as testing endpoints
Epigenetic clocks remain a powerful tool in the science of rejuvenation. In the short term, researchers believe their best use is as a measuring tool, a kind of epigenetic yardstick that determines whether other interventions are successful. Although there are outstanding questions about how we define aging, how we measure rejuvenation, and how this could unfold economically, epigenetic clocks are “a true revolution,” Gladyshev says. He calls Horvath “a hero” and adds that the clocks represent a leap forward in quantifying the intricate process of aging.
Longevity research could speed ahead, no longer reliant on death as a primary endpoint.
When it comes to aging research in humans, epigenetic clocks could be a tool that helps quantify a treatment’s effectiveness while subjects are still alive. In other words, if epigenetic clocks become sophisticated enough that the FDA accepts them as surrogate endpoints, it would allow researchers to quickly demonstrate a drug’s efficacy in mere months by measuring methylation—as opposed to waiting years to see how the drug affects survival. Longevity research could speed ahead, no longer reliant on death as a primary endpoint.
Picture this: Your doctor wants you to take vitamin D. Instead of simply popping a daily pill and waiting a year or more to follow up, you mail order a test kit that tracks your epigenetic age before you start the supplement. After a few weeks or months, you test again, checking to see if your biological age has decreased. At present, a blood test can check your vitamin D. But no test can determine whether your exercise program or, say, a new experimental drug that targets and destroys senescent cells, could actually be extending your lifespan. And such an endpoint is desperately needed to legitimize the work of dozens of startups that hope to build on Horvath’s discovery.
Partial cellular reprogramming
One of the most promising—and potentially most dangerous—therapies that could harness epigenetic clocks as surrogate endpoints is partial cellular reprogramming. It’s a technique that’s a bit like taming a wild horse. If you can calm the animal long enough to slip into the saddle, you could be in for a speedy ride. If you falter, a swift hoof to the ribs is more likely.
Yamanaka factors are four specific proteins that regulate the transcription, or expression, of four specific genes. Stem cell researcher (and later Nobel laureate) Shinya Yamanaka discovered that altering these four proteins could convert a differentiated cell, such as a muscle, liver, or kidney cell, to a fully undifferentiated cell, similar to an embryonic stem cell. They are called induced pluripotent stem cells.
Like other epigenetic breakthroughs, induced pluripotent stem cells have a drawback: They’re really, really good at forming ugly, cancerous tumors called teratomas. Similar to embryonic stem cells, the induced pluripotent stem cells have the potential to differentiate and become anything.
“The problem with stem cells is if you take them out of context, you remove the controls, the feedback mechanism,” Horvath says. The result is “chaos, chaotic growth.” That means a high rate of cancer, which is a major safety risk.
But scientists had another breakthrough. It turns out that specialized cells can be partially turned back. This process returns cells to a younger state that avoids dedifferentiation, so there’s less risk of causing cancer. Thanks to epigenetic clocks, scientists have a benchmark, albeit an imperfect one, of how well this partial reprogramming works. It’s a technology still in its infancy. But it showed promise in mice and frogs, and Chandra recently applied it to human cells, as well.
“We could actually show that these cells maintain the somatic identity in these early days after reprogramming, but the epigenetic age decreases,” he says. And that somatic, or specialized identity, is important to maintain because it would allow cells to be reintroduced in people after being altered. Imagine in a futuristic and hypothetical world modifying cells from a damaged organ, like a heart, for instance, then reinjecting them back in the donor.
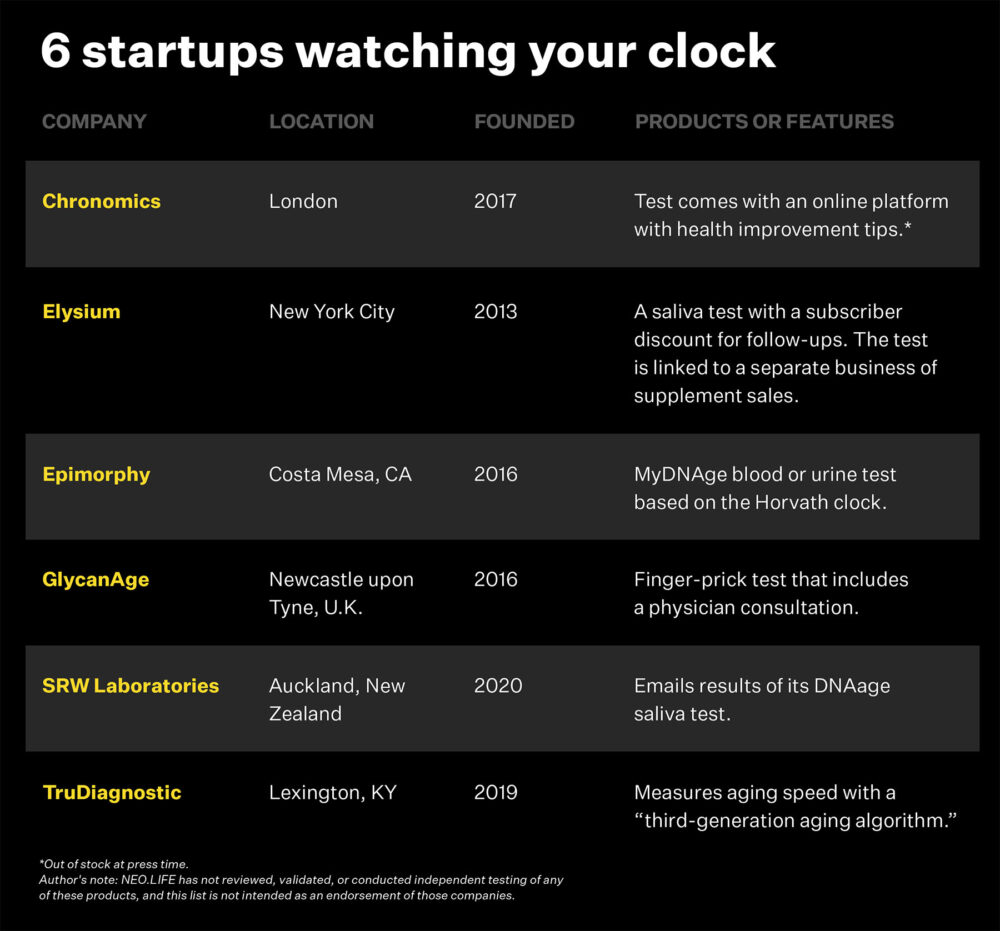
The aging quandary
And there’s one final caveat Horvath shares, a kind of scientific quandary. “The question is, why do we age? Well, maybe there’s a purpose to it, to actually prevent malignant transformation. Maybe we age to suppress cancer risk. And so then, by reversing it, maybe you actually increase cancer risk.”
And the potential of epigenetic clock tinkering is counterbalanced by consumer risks, too, according to University of Montreal bioethicist Charles Dupras. Startups, he says, have a commercial interest in selling subscription-based tests that may not be accurate enough to serve as a useful marker to buyers, particularly if they’re retesting every few months. Every epigenetic clock, after all, has some margin of error.
As to current at-home epigenetic tests, Dupras says their best use is “for purely recreational purposes,” cautioning that buyers should wade through each lab’s policies for privacy practices and other safeguards. “Some of them are really lengthy and hard to understand… there aren’t standards of practice for this kind of thing.” He also points to concerns about the potential misuses of epigenetic data—imagine health insurance premiums matched to epigenetic measurements in a dystopian future.
For now, Horvath is working with bold new funding in a brave new field, the proverbial kid in a candy store of his own making. His research has been cited nearly 90,000 times. And he seems to view even his peers not as competition but as deputies of a shared mission to figure out a place for epigenetic clocks in the race to help humans live longer, healthier lives. As he considers their work, he energetically ticks off prime candidates for rejuvenation, refusing to rank them: interrupted cellular reprogramming via Yamanaka factors; senolytic drugs that clear “senescent” cells that don’t die when they should; calorie restriction mimetics, or medications that mimic the impacts of calorie restriction; autophagy, which involves clearing out damaged proteins; and parabiosis, or linking the circulatory systems of two organisms.
For the moment, Horvath is focused not just on a fantastic, if unspecified, future, but also the boring basics. After explaining why Altos Labs higher-ups prefer to use the term “cellular resilience” to “anti-aging,” he notes one clear outcome of all his groundbreaking work with a laugh. “In people who eat vegetables, their methylation estimate of mortality risk is quite reduced,” he says.
While we wait for the technologies that could save us from frailty, untimely death, and dementia, a little salad wouldn’t hurt.